World
How Wildfire Defines the World
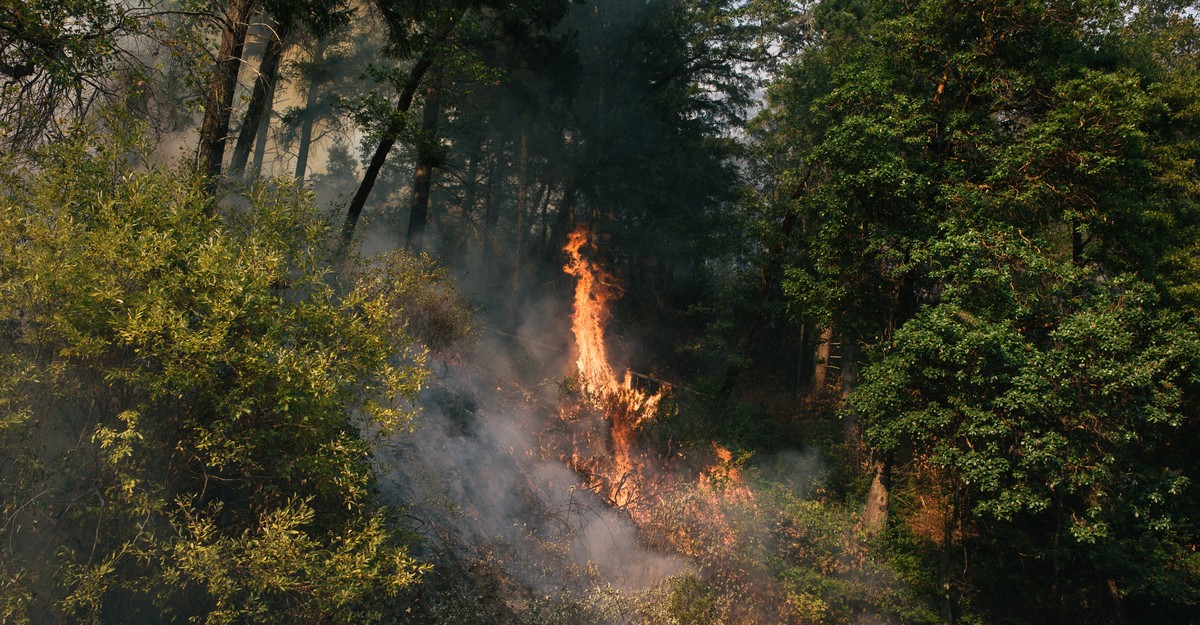
Perched on a densely forested hill crisscrossed with narrow, winding, often unsigned roads, Frank Lake’s house in Orleans, California, is not easy to find. On my way there one afternoon in late October, I got lost and inadvertently trespassed on two of his neighbors’ properties before I found the right place. When Lake, a research ecologist for the United States Forest Service, and his wife, Luna, bought their home in 2008, it was essentially a small cabin with a few amenities. They expanded it into a long and handsome red house with a gabled entrance and a wooden porch. A maze of Douglas firs, maples, and oaks, undergrown with ferns, blackberries, and manzanitas, covers much of the surrounding area.
“This is a feral orchard,” Lake said as he showed me around, weaving among slender-trunked trees and sprawling shrubs. He was wearing cargo pants, thick black boots, and a camo-print beanie. “This is an old place that Karuk managed.” Lake, who is of mixed Indigenous, European, and Mexican heritage, is a descendant of the Karuk, a native people of northwestern California and one of the largest tribes in the state today. Some of his family members are also part of the Yurok Tribe, which is indigenous to the same region. Lake grew up learning the history and culture of both peoples.
A little ways ahead, we reached a grove of moderately large oak trees. Here, the forest floor was mostly free of vegetation, charred black in places, and littered with acorns. Since 2009, Lake, who is a certified firefighter, has used chain saws, propane torches, and drip torches to strategically thin and burn this particular half acre. Over the years, the controlled burns, or prescribed burns as they are often called, have removed the smothering underbrush, reduced the number of trees, and provided the remaining oaks with much more light and space, creating an orchard similar to those Lake’s ancestors would have managed.
Fire has also kept pests in check. Every year, weevils and moths lay eggs on or within acorns, which their larvae proceed to devour. Periodic low-level fires spare the trees but kill a portion of the pests’ pupae buried in leaf litter and soil, preventing them from ruining the following year’s crop. Like many Indigenous peoples in the area, Lake’s family and friends continue to use acorns to make flour, bread, and soup.
“How do you know which ones are best?” I said, scanning the hundreds of fallen acorns around our feet.
“Look for silvery-white ones,” Lake said. He rummaged through the leaf litter, his fingers moving too quickly for me to follow. “Okay, here we go. Brown top bad. White top good.” He showed me several large acorns with neat white circles on their rounded ends. “A stain on top usually represents that there’s a bug hole or injury. When it’s clear, then the inside is usually good too.” Lake cracked open an acorn and split it in half lengthwise. The flesh was smooth and creamy white with a tinge of yellow, like French vanilla. He turned it this way and that, as if he were inspecting a jewel. “That is a perfect acorn,” he said. We explored more areas of the grove as he continued: “This is what traditional management and food security looks like … And this is climate adaptation. If someone tosses a cigarette on a hot summer day and a wildfire comes through here, this clearing will be a barrier between the fire and my home.” Fire had created what he called “a place of safety.”
Wildfires in many parts of the world are becoming more frequent, intense, and disastrous. In the context of anthropogenic global warming, the concept of a discrete “fire season” is unraveling because devastating blazes can now happen at any time of the year. Yet the horrors of the current wildfire crisis all too easily obscure an essential truth: that fire is not always destructive. Fire can be beneficial. Fire can be life-giving. In fact, fire is a product of life. Over the past 400 million years, wildfire has become a vital component of the vast living system we call Earth. Without it, forests and grasslands as we know them would not be possible, the level of atmospheric oxygen might be far less stable, and much of human history would never have happened.
For the first few billion years of Earth’s history, wildfires did not exist. Fire requires three ingredients: fuel, oxygen, and heat. In Earth’s youth, there were many sources of intense heat and plenty of sparks—lightning, volcanoes, rockfalls—but hardly any free oxygen or dry and combustible matter. By 600 million years ago, photosynthetic cyanobacteria and algae had raised the amount of oxygen in Earth’s atmosphere to somewhere between 10 percent and half of its current level—a monumental change but not quite sufficient for fire. The creation of a more familiar atmosphere required a second revolution: the greening of a new Earthly domain.
About 425 million to 500 million years ago, the first land plants evolved. Cooksonia was a tiny, moisture-loving plant with spore-bearing structures that resembled the toe pads of a tree frog. Baragwanathia longifolia’s undulating branches, densely packed with slender leaves, gave it a hirsute, tarantula-like appearance. And the 23-inch-high Psilophyton dawsonii, with a rather sophisticated vascular system for its time, looked like a primordial cousin of dill. Over the next several hundred million years, terrestrial plants of all kinds profoundly altered the planet, accelerating the water cycle, turning obdurate crust into supple soil—and pushing the level of atmospheric oxygen to new heights.
The process by which this happened was not as simple as plants exhaling oxygen into the air. The great majority of oxygen that plants breathe out is used up by other organisms in a perpetual cycle. In order to grow, plants absorb carbon dioxide, use it to build their tissues, and release oxygen as a waste product. Animals, fungi, and microbes eat and decompose plants, using oxygen in the process and exhaling carbon dioxide. Not all plant material is consumed or decomposed, however. A fraction is buried relatively intact in lakes, swamps, landslides, and seafloor sediments. The oxygen that animals and decomposers would have used to break down those absentee plants remains in the atmosphere, having escaped the usual cycle. This leakage began in the ocean about 2.5 billion years ago with photosynthetic cyanobacteria, but it accelerated when plants evolved on land. Bit by bit, across the Paleozoic era, excess oxygen accumulated.
Along the way, fire became routine. The charred remains of 430-million-year-old plant fragments are the earliest evidence of wildfire. Charcoal has been present in the fossil record ever since. From the late Devonian onward, many plants adapted to fire’s recurring presence. They evolved thick, flame-resistant bark, succulent leaves, and resilient tubers that resurrected themselves in charred soil. Some plants even came to depend on fire to reproduce: Certain pine trees have cones sealed by resin that melts in the heat of a wildfire, releasing seeds into fertile ash; smoke seems to stimulate germination in some plant species; and a few flowering plants burst into bloom only after a blaze.
In tandem, fire adapted to life. “Fire cannot exist without the living world,” the fire historian Stephen J. Pyne writes in Fire: A Brief History. “The chemistry of combustion has progressively embedded itself within a biology of burning.” Wildfires coevolved with the very ecosystems that made their existence possible. The outcome is known as a fire regime: the typical frequency, intensity, and duration of wildfires in a given region. If fire is itself a kind of music that results from the interplay of life and environment, then a fire regime is a tune or theme that recurring wildfires and their particular habitat compose together.
Once fire became a frequent occurrence in the Earth system, an entirely novel evolutionary path emerged: the chance that one or more creatures might learn to control it. At some point, possibly 1 million to 2 million years ago, our ancestors began to do just this. Archaeological evidence suggests that humans were routinely maintaining fires by about 400,000 years ago.
Fire was warmth when there was no sun and light when it was not day. An evening campfire became a focal point of conversation and storytelling. A torch or an oil lamp turned the formerly dark contours of a cave into a canvas for myth and memory. A combination of hunting and cooking with fire allowed our species to evolve and nourish much bigger, denser, and hungrier brains with nearly three times as many neurons. Fire is arguably the single most important catalyst of human evolution—the furnace behind our intelligence, technology, and culture.
Strategically burning the environment is undoubtedly an ancient practice, but its exact origins are lost to unrecorded history. What is certain, however, is that whenever Indigenous peoples began to experiment with controlled burns—not just in North America but in Africa, Australia, and Asia too—they did so within the context of existing fire regimes that had developed over many millions of years. Over millennia, humans became co-conductors of fire’s ecological rhythms. Eventually, we would alter them more drastically than any creature before us—sometimes to marvelous effect, sometimes with dreadful consequences.
The day after meeting Frank Lake at his property, I ventured northeast of Orleans, past Somes Bar, and into Klamath National Forest, near an area known as Rogers Creek. Moss pillowed every rock, trunk, and stump. Wisps of pale lichen hung along the length of every branch, as though the trees were antique chandeliers caked in melted wax. The stout smell of wet soil and rotting leaves flavored the air, muddled with their near opposites: the scent of woodsmoke and ash.
Dozens of people dressed in flame-resistant clothing—mustard-yellow shirts and pine-green pants—paused along a forest service road to adjust their hard hats, strap propane tanks onto their backs, and test the torches connected to them: long, thin metal rods with a stream of flaming gas at one end. Although they were all firefighters, they were not there to extinguish anything. They had come to burn. A diverse group of conservationists, paramedics, members of local Indigenous communities, and pyrophiles, they had traveled from near and far to participate in a program known as TREX: prescribed fire training exchanges. Founded in 2008 by the U.S. Forest Service and the Nature Conservancy, TREX teaches people how to use controlled burns to benefit ecosystems and reduce the chance of severe wildfires.
The firefighters—some of whom prefer to be called firelighters—moved carefully down steep slopes into the midst of the forest, searching for large piles of branches and brush, which crews of foresters had cut and stacked in the preceding months, covering their centers with wax paper to keep them dry. When a firelighter found a brush pile, they would push their torch into its heart and squeeze a lever or turn a knob to increase the flow of gas, scorching the pile’s interior with a fierce orange flame.
At first, some of the piles seemed too wet to burn properly. Although they spewed plumes of smoke like volcanoes stirring from slumber, they did not erupt in flame. A little rain is beneficial for pile burning, as it prevents fires from becoming too big and hot, but too much moisture defeats the purpose. The forest ecologist and firefighter Michael Hentz explained that the piles needed time to burn and dry from the inside out before catching fire in their entirety. As the day progressed, more and more piles began to burn, sometimes so vigorously that they lofted ash and embers high above us. Soon the whole forest seemed to glow and crackle within shifting layers of fog and smoke. Although I knew that these fires were intentional, the sight of them still provoked some deeply embedded survival instinct—a stubborn feeling that something was wrong. It was strange to see the forest on fire. It was beautiful too. Surveying the many heaps and rings of wood with flames leaping from their centers, I felt like we had stumbled into a colony of phoenix nests.
“This is one of the most important steps in reintroducing fire back to this mountainside,” Zack Taylor, the burn boss and one of the key organizers of the day’s events, told me. The 50 acres on which they were burning, he explained, were populated with a mix of tan oak, black oak, canyon live oak, big-leaf maple, madrone, and a surfeit of spindly Douglas firs. “The ecological trajectory we want is one in which we have less conifers and more healthy hardwoods,” he continued. “They’re an important cultural food source, and they have a lot of value for wildlife, but they’re lacking on the landscape because of a hundred years of fire exclusion.”
When Frank Lake was a boy, TREX was many decades away from existing, Indigenous burning traditions were still sometimes prohibited by law, and prescribed burns in the West were uncommon. After earning a Ph.D. in environmental science from Oregon State University, Lake became a key figure in collaborations between the Forest Service and Indigenous tribes, as well as a champion of the growing movement to return fire to western North America. Thanks in large part to advocacy by Indigenous leaders, both federal and state government agencies are more and more open to using prescribed fire to reinvigorate ecosystems and reduce the likelihood of disastrous megafires.
I asked Lake what he envisions for the future. “I want to scale up,” he said with typical fervor. “If my gold standard is my half-acre orchard, we should have 50,000 acres of it. I have learned this Western system of sound, credible science. I’ve been able to use that to demonstrate that Indigenous practices can fulfill desired objectives for carbon sequestration, climate resilience, and the mitigation of severe wildfires. What I do is no longer questioned the way it was before. You serve by example.”
When fire first became part of the Earth system, it was highly volatile. The rhythms that characterize modern fire-adapted ecosystems took hundreds of millions of years to form. Earth’s earliest wildfires may have been fitful and erratic, flickering among the amphibious flora of fens and bogs. In contrast, during the Carboniferous, between 275 million and 375 million years ago—when atmospheric oxygen levels were at their peak and giant dragonflies soared through the air—fires were frequent and rampant, incinerating even lush vegetation. For a long time, oxygen levels, and the frequency and intensity of wildfires, fluctuated widely.
About 200 million years ago, however, something appears to have changed: The amount of oxygen in Earth’s atmosphere began to stabilize, remaining within a relatively narrow window around 18 percent. Fires cannot sustain themselves if the atmosphere contains less than 16 percent oxygen; conversely, if oxygen exceeds about 23 percent, wildfires are much more likely to blaze out of control, and essentially anything that isn’t drenched or submersed in water becomes flammable. In the past 55 million years, atmospheric oxygen has been more stable than ever, hovering around 21 percent, which is high enough to support occasional wildfires and an incredible diversity of complex, fire-adapted life, yet not so high that any stray spark will ignite an unstoppable inferno. Scientists have long struggled to explain this remarkable equilibrium. In the past couple of decades, they have begun converging on a possible answer: the coevolution of fire and life.
The geoscientist Lee Kump was one of the first scientists to formally publish a theory of this particular planetary balancing act, which was further developed by the Earth-system scientist Tim Lenton. The gist of their idea is that the level of oxygen in the atmosphere is regulated by the overall productivity of terrestrial plants. When land plants thrive, there is an abundance of plant tissue on the planet, and an even higher-than-usual amount of that carbon-rich organic matter is buried before it is eaten or decomposed, strengthening the mechanism by which oxygen accumulates in the atmosphere. If oxygen levels rise too high, however, wildfires become more intense and frequent, destroying immense tracts of vegetation, hindering the process of oxygen accumulation, and bringing the oxygen level down again. Although this feedback loop is not yet textbook science, a growing cadre of scientists think that it has stabilized the amount of oxygen in Earth’s atmosphere for 50 million years.
Such feedback invokes one of the most provocative ideas in the history of Western science: the Gaia hypothesis. Developed by the British scientist James Lovelock and the American biologist Lynn Margulis, the Gaia hypothesis characterizes Earth as a giant, living, self-regulating entity. When the original version of Gaia rose to prominence in the late 1970s, some of its most controversial tenets were that life controls the global climate in order to benefit itself and that the Earth system as a whole actively “seeks an optimal physical and chemical environment for life on this planet,” as Lovelock initially phrased it. As Earth history shows us, that is not quite true. To the contrary, many forms of life—as varied as microbes, trees, and bipedal apes—have caused or exacerbated some of the worst crises in Earth history. And there is no single “optimal” state of the planet that would suit all of the manifold and wildly diverse types of life that have existed in the past 4 billion years. In general, though, given enough time and opportunity, life and environment seem to coevolve relationships and rhythms that ensure their mutual persistence. There is nothing teleological about this. Such persistence is not designed or planned. It is the outcome of ineluctable physical processes that are distinct from, but related to, the processes that govern the evolution of species.
All complex multicellular organisms have evolved numerous ways to maintain homeostasis—to preserve a steady state of physical and chemical conditions essential to their continued existence. All complex organisms are also chimeras: Their genomes are patchworks stitched with genes introduced by viruses and pilfered from other species; some of the organelles in their cells were once free-living bacteria subsumed in the emergence of multicellular life; their bark, fur, or skin teems with trillions of microbes, competing, cooperating, and multiplying in secret societies. Any individual plant, fungus, or animal is, in effect, an ecosystem. If such composite creatures can evolve homeostasis—a point about which there is absolutely no disagreement—then perhaps an analogous phenomenon, which science does not yet fully understand, occurs at the scale of forests, grasslands, coral reefs, and other ecosystems.
Ecosystems might not compete and reproduce the way organisms and species do, but some scholars have proposed that they should be regarded as living entities capable of self-regulation and evolution. The coevolution of the organisms and habitats that compose a given ecosystem influences how that system changes over time. An ecosystem, then, does not evolve passively; it effectively changes itself through inevitable feedback loops—at least to an extent. Although the particular species and habitats within these systems shift dramatically over time, the fundamental relationships that define them, the cycles and webs that bind prey and predator, flower and bee, leaf and flame, and the physical infrastructure that life creates—the rich soils, webs of roots and fungi, reefs, and ocean sediments—typically persist or, if they are demolished, regenerate in some form. Networks of species that happen to help sustain the system as a whole will be favored, whereas those that undermine the system to the point of collapse will ultimately eliminate themselves, even if they profit in the short term. The most resilient ecosystems—those best able to adapt to challenges and crises—will survive the longest.
Perhaps this phenomenon of persistence extends to the planet as a whole—not an intention to persist but a tendency; not an imperative but an inclination. Whether cell or cetacean, prairie or planet, living systems find ways to endure.
This article has been adapted from Ferris Jabr’s new book, Becoming Earth.
When you buy a book using a link on this page, we receive a commission. Thank you for supporting The Atlantic.