World
The World’s First Nuclear Clock Could Unlock the Universe’s Dark Secrets
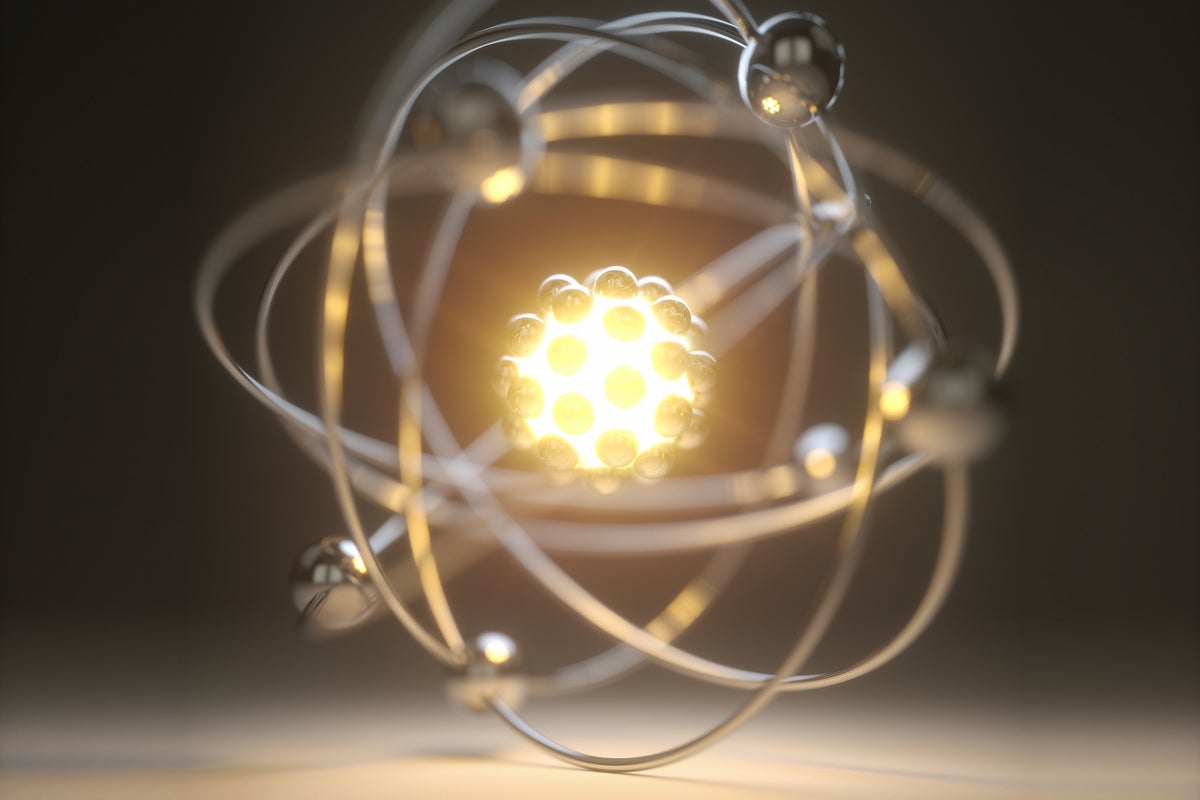
To measure time, you need a constant rhythm. For eons, the regular movements of the sun and moon have set the pace for all of life on Earth. But across millennia, humans have sought and found more controllable and precise methods, such as the swing of a pendulum or the rates at which water flows and incense burns. Today most electric clocks or watches measure the steady vibrations of quartz crystals.
But even the best pacemakers drift; in one form or another, creeping chaos eventually corrupts the order of any timekeeping system. A month’s worth of varying temperatures and movements, for example, can be enough to knock a quartz watch off its mark by 15 seconds. Questing for a truly constant “tick,” scientists have zoomed in on the atomic world, where the minuscule scales and unchanging physical properties of atoms allow time to be sliced into smaller, more stable increments. The resulting atomic clocks that set official international times today are astonishingly robust, losing only about one second every 100 million years.
For decades, physicists have been trying to escape clock-contaminating noise by zooming in even further, past an atom’s outer electron shells and into the far smaller, more tightly wound atomic nucleus. Just last week they finally succeeded. The world’s first nuclear clock, created in Boulder, Colo., by an international team of scientists, was detailed in a paper published in Nature. Although this particular nuclear clock is not yet more accurate than its best atomic partner, the technology could soon shatter precision timekeeping records and vastly improve GPS and deep-space navigation.
On supporting science journalism
If you’re enjoying this article, consider supporting our award-winning journalism by subscribing. By purchasing a subscription you are helping to ensure the future of impactful stories about the discoveries and ideas shaping our world today.
And making such sharp measurements within an atom’s nucleus might just give physicists access to vast, unexplored realms of the physical world. This “will really open a new window into looking at the fundamental laws of nature,” says José R. Crespo López-Urrutia, a physicist at the Max Planck Institute for Nuclear Physics in Heidelberg, Germany, who was not involved in the new research but co-authored a companion article that was published in the same issue of Nature.
At their core, atomic clocks and nuclear clocks work using the same principle. Atoms can become “excited” when they’re hit by photons packing just the right punch—that is, waves of light at a very specific energy level, or frequency. Which tiny range of frequencies will do the trick depends on the type of targeted atom, but it remains the same for all atoms of a particular element. “An atom of cesium or an atom of sodium is the same everywhere [in the universe], or at least that’s what we believe,” Crespo López-Urrutia says.
In the 1940s scientists realized this cosmic constancy could be used to measure time without the chaotic noise associated with other physical clocks. Photons travel as waves, and their frequency is simply a measure of how many wave cycles pass a given point per second. So you can turn a laser beam with a known, fixed frequency into a clock by counting its waves to determine time’s passage. The best way scientists have found to do this is to excite an atom by hitting it with an ultraprecise laser using the exact frequency of the beam as a constant measurement of time.
Since the first atomic clock was built in 1949, this process has only gotten more sophisticated. The most precise and accurate atomic clock in the world, the ultracold strontium clock at JILA in Boulder, is like a stopwatch that can count the billionths of a nanosecond, or 18 digits past the decimal point. It loses only one second every 40 billion years.
But physicists have long dreamed of something even better. What if they could excite not entire atoms but the nuclei deep inside? Nuclei contain 99.99 percent of an atom’s mass but are truly minuscule; if an atom were the size of an American football stadium, its nucleus would be only as big as a marble. Exciting something so tiny and heavy requires thousands or millions of electron volts of energy, usually in the form of photons that are precisely tuned to incredibly specific frequencies. This difficulty is exactly why a nuclear clock could drift less and “tick” more times per second than an atomic clock. But unfortunately the demands of this task exceed the capabilities of any tabletop laser.
There is one exception, however: the rare radioactive isotope thorium-229. This isotope requires only about eight electron volts of energy to jump to an excited state, and physicists have no idea why. “Its [transition energy] is so low that nuclear physicists actually don’t know what to do with it,” says co-author Thorsten Schumm, a physicist at the Vienna University of Technology.
This exception provided a golden opportunity for nuclear clock builders. But while thorium-229’s transition energy was technically within reach, they had to find it first. Only a minuscule range of energies could excite the nucleus, and narrowing down that range required building brand-new laser systems and many years of clever trial and error by physicists around the globe.
“It’s this ‘needle in a haystack’ problem—although finding a needle in a haystack is much easier than what we did, in terms of orders of magnitude,” Schumm says.
The researchers also had to find a way to hold the thorium-229 atoms still. While many atomic clocks suspend single atoms in a vacuum using electromagnetic fields, physicists building a nuclear clock knew they’d have a better shot at success if they could hit many nuclei at once. Schumm’s team embedded tens of thousands of thorium-229 nuclei in transparent crystals, allowing more nuclei to be hit by the lasers at one time and increasing the probability that some would transition to their excited state.
In just the past year all of these necessary discoveries finally began converging. In May Schumm’s team shipped the thorium crystals to the Boulder lab running the ultracold strontium clock. Researchers there had developed an advanced laser system that could narrow the range of remaining possible frequencies down to a precise answer. This laser system could also sync up the nuclear “ticks” with the atomic clock, allowing the atomic clock to stay on track with nuclear time.
The Boulder team shot its special laser system at the crystal, sweeping through different frequencies. A faint telltale glow from the excited thorium would be the only sign of a successful nuclear transition. For weeks, however, the researchers saw nothing.
“It was close to midnight when we saw the first indication of the signal,” says Chuankun Zhang, a Ph.D. student studying laser physics at the University of Colorado Boulder. “Nobody could sleep after the experiment.” Instead the team spent those sunless early-morning hours verifying its results. By around 4 A.M., the conclusion was crystal clear: Zhang and his team had successfully excited the thorium-229 nuclei and synced their frequency measurements with JILA’s atomic timekeeper, creating the world’s first nuclear clock. Currently it falls short of the world record for precision because it only reads out 12 digits of a frequency measurement compared with the strontium clock’s 18.
“This first realization is not about ‘Oh, they got only 12 digits.’ The first realization is ‘Oh, they got this thing to run,’”Crespo López-Urrutia says. While technical challenges remain, mostly involving the laser system, “people are confident that they can be overcome in a few years,” and then the nuclear clock will overtake the atomic clock in preciseness and accuracy. Measurements reaching to 20 or even 21 digits may be possible, he says.
“It’s beautiful work,” says physicist Eric Hudson, whose team at the University of California, Los Angeles, has also been working to narrow down the transition frequency and grow thorium crystals. Hudson is most excited about the team’s creation of a crystal-based, or “solid-state,” clock, which trapped the nuclei in place rather than levitating them with electromagnetic fields. “This could lead to a much more portable and robust clock that could come out of the lab into the real world,” he says.
These portable nuclear clocks could find their way onto GPS satellites or spacecraft navigation systems. GPS satellites triangulate a device’s location by measuring tiny differences in a signal’s transmission time. The technology is currently accurate down to a few meters for nonmilitary applications. That’s too inaccurate for autonomous cars, Schumm says, or for positioning technology that could help people who are blind or have low vision navigate. “There’s an urgent need to get positioning to centimeter- or millimeter-level because then there’s a qualitative change.”
And on a more fundamental level, nuclear clocks provide an entirely new way to study the unseen forces and particles that surround us. The frequencies measured by atomic clocks arise from the jostling of electrons, meaning they are governed by the well-understood electromagnetic force. But the ticking of a nuclear clock comes from a comparative terra incognita, the mysterious domain of the strong force that binds nuclei together. Physicists generally assume that these two forces remain constant over time, but they haven’t been able to rigorously test whether that is true, Zhang says. However, by comparing the outputs of an atomic and a nuclear clock, they can, in principle, track whether these two forces are truly unchanging.
It’s also possible that this nuclear probe could reveal something about dark matter, which remains elusive despite being 85 percent of the matter in the universe. “Our difficulty with dark matter is that it doesn’t interact with what we know, so we don’t have [successful] detectors yet,” Schumm says. But if dark matter interacts with the nucleus differently than it does the atom as a whole, those differences might show up in comparisons between nuclear and atomic clocks.
“All those forces which are yet not well explained, or for which the origin is unknown, could appear in the comparison of frequencies of clocks,” Crespo López-Urrutia says. If their pace changes relative to one another, scientists in search of a steady timekeeper might instead have discovered that there’s no such thing after all.